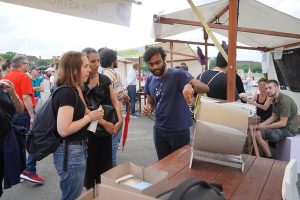
Long night of Astronomy 2023
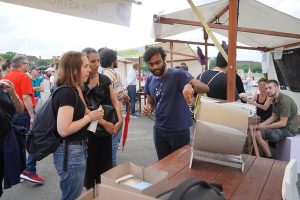
Bringing Physics and Astrophysics to the people
Once upon a time in China, Astronomers saw something odd in the sky. There was a star that didn't belong in that part of the sky. Was it a new star? They didn't know. What they did know was that it wasn't there before, so they named it "Guest star" \cite{Cosmos}. It was the year 185 A.D. This guest star would last for another 8 months and then would slowly fade away. This was the first observance of a guest star \cite{Zhao_2006}. Subsequently, many guest stars were observe in years to come .
Figure 1.1 : RCW86 — Nasa/JPL-Caltech/WISE Team — Public do
Today we know that these "Guest stars", as described by Chinese Astronomers were of many kinds. Some of them were comets, while some of them were giant explosions in space. Astronomers were able to determine that the guest star visiting Chinese Astronomers was actually an explosion called Supernova. The shockwave from the supernova observered by the Chinese astronomers is still visible today and called the RCW86 \cite{Zhao_2006}. A recent picture taken from European Space Agency's XMM-Newton Observatory and NASA's Splitzer Space telescope of the RCW86 is showed in figure 1.1.
The RCW86 is approximately 75 thousand trillion kilometers away from the earth. The explosion is about 80 trillion kilometers big today. If the artifacts of the supernova was visible to the naked eye, it would occupy an area almost as big as the moon. Clearly this had to be a very big explosion to be this big today.
Figure 2.1: First photographic plate blackened by ”Becquerel rays”. It was placed under a uranium salt on 26.02.1896 — by Henri Becquerel — source: wikimedia
It was the year 1896. Henri Becquerel, a french Physicist had just finished publishing a paper on how Uranium crystals absorb sunlight and re-emit it like a phosphorescent material. A phenomenon similar to the glow in dark stars and planets we had on our roofs as a child or the glowing dials on our wristwatch. For this experiment, he had used Uranium salts which were wrapped in photographic plates and kept them in the sun. Whenever he did this experiment, he noticed the emitted rays from the salts would leave their imprint on the plates. These mysterious rays were named as "Becquerel rays".
After reporting his results at the Academy of Sciences, he wanted to conduct more observations and collected more data on this phenomenon, However, the Parisian weather in February was not on his side. It was cloudy. Without the sun, the Uranium salts would not have enough stored energy to re-emit Becquerel rays. Disappointed by this, he kept his uranium crystals and the photographic plates in his desk for the next few days until the weather would eventually clear up. On March 1, he decided to open the drawer. Since the Uranium was not exposed to sunlight, he expected to see a weak image, perhaps stored energy that was slowly being emitted by Uranium, so he developed the plates. He observed that the developed plates had strong imprints of the Becquerel rays even when the salts were not exposed to sunlight [Figure 2.1]. This was the discovery of radioactivity.
The radioactive Uranium contained in the salts can be extracted and concentrated to make atomic weapons or be used to generate enough energy to power cities. What Becquerel discovered inadvertently was a process called Nuclear fission that released radiation. Becquerel would later be awarded a Nobel prize in Physics for this discovery and the unit of measurement of radioactivity would subsequently be called "Becquerel", just like the unit of length is "Metre".
An atom consists of Protons, Neutrons and Electrons. The Protons and Neutrons are packed in the centre and make up the nucleus. They are the "building blocks" of the nucleus. These are held together by a strong nuclear force, which acts as cement and holds the Protons and Neutrons together. The strong nuclear force acts like a bar magnet. Just like a bar magnet pulls another bar magnet through an invisible force. The strong nuclear force holds the particles together through something physicists call a "field". This force cannot work at large distances unlike a magnet. For the strong force, this is just a thousand trillionth part of a meter or $10^{-15}$m.
Figure 2.2: Illustration of Hydrogen, Deuterium and Tritium, Source: wiki- media
An atom of Hydrogen consists of 1 proton, while that of Helium consists of two protons and two neutrons. There are also other "isotopes" or types of hydrogen with neutrons. These variations don't change the property Hydrogen and are called Isotopes. The Isotopes of Hydrogen are Deuterium and Tritium. They contain 1 and 2 neutrons respectively in addition to one proton. Oxygen consists of eight protons and eight neutrons.
Uranium, or more specifically Uranium-235 ($^{235}$U) has a massive nucleus. It holds 92 protons and 143 neutrons. If we add one more neutron to this already massive nucleus, we get $^{236}$U. This is a very unstable form of Uranium and the nucleus breaks into smaller more stable nuclei. The smaller nucleus produced from this process is known as a daughter nuclei and the process is known as Nuclear fission (Figure 2.3 ).
A by-product of this Nuclear fission are 3 neutrons (Figure 2.3). These three neutrons can hit other $^{235}$U and break them too. This causes a chain reaction. Since Nuclear fission breaks up the strong nuclear force. It also releases an immense amount of energy and is therefore used as a fuel in Nuclear fission power plants and in Nuclear weapons.
The instability of $^{235}$U makes it sound dangerous, as if, we do not know anything about it. This is somewhat true. For example, we do not know when a particular nucleus would break up and form daughter nuclei, but we do know that when we have 1000 atoms of it, it would take 700 million years for us to have 500 atoms left. This is usually expressed in a term called the "half life".
Figure 2.3: Break up or Uranium 236 into Krypton 92 and Barium 141, source: wikimedi
If the nucleus can break up into daughter nucleus, can we combine two smaller nuclei and make a bigger one? Yes, we can indeed combine two the nuclei. We can take two hydrogen atoms and combine them to make Deuterium. Artificially, we can do this inside a fusion reactor. We can also take two Deuterium atoms and combine them to make Helium. This combining process is called Nuclear fusion.
Astronomers believe that this is the process that takes place inside all stars like ours. As we shall see in section 3.3. A star is basically a big ball of Hydrogen and Helium held together by gravity. The gravitational force in the centre of the sun can get so strong that Nuclear fusion can occur on its own due to the pressure. This generates Helium. As the star ages and it runs out of Hydrogen, we can get other phases of star where Heavier elements such as Oxygen, Nitrogen and other heavier elements up till Iron can be produced. Astronomers believe that all the heavier elements that make up our trees, houses, food and even the carbon in our body come from stars. We are made of star stuff.
Say you are driving at night on a dark highway with no lane markings and you see a faint headlight up ahead. What can you tell about the vehicle from just the lights? Well, the first one is pretty obvious, if it is a single light source, then it more likely that it is a two-wheeler than a four wheeler. If the light is yellow, rather than white, then it is probably an older car. Since newer vehicles are required by law to have only white light. In our case, the lamp is yellow and single source. From our daily experience of seeing cars and motorbikes at night, we know approximately how bright yellow headlights are, since the lights are very dim, we can assume that the motorbike is pretty far away, so we don't have to worry about slowing down our car, until the light gets brighter.
Astrophysicists use the same "intuition" to find distances and type of stars in space. The colour of the star helps us know the type of star and the brightness tells us how far it is, however, they do this mathematically rather than depending on intuition. Say you have a star and you know its brightness from a distance of 1 km (a random distance that I selected, this could be 5km or even 100km, it doesn't matter as long as it is measurable) This tells us how bright this object would be from 50 km or from earth. The brightness from a known distance of 1 parsec is known as the absolute magnitude of a star. Parsec is a distance measure that Astrophysicists use to measure distances in space. Since the universe is so big, using metres or even kilometres is not feasible. One parsec is about 31 trillion kilometres. The nearest galaxy, the Andromeda galaxy is about 778,000 parsecs away. The brightness observed from an object in space from Earth is called the apparent magnitude. \cite{Cosmos}.
Each person on Earth has a different fingerprint. Even twins, who share the same physical features, do not share their fingerprint. Similarly, elements such as Hydrogen and Oxygen have a unique feature too. The "fingerprint" is the light they emit and absorb. If you have ever used a prism, you may have seen the rainbow created by the sunlight. However, if you take a microscope and look at this rainbow carefully, you will see that some of the colours are missing. These missing colour "lines" are the fingerprint of the sun. Each element has a different fingerprint. The missing lines in the light of the sun tells us what the sun is made up of. This is how Astrophysicists study the composition of planets and stars without actually sending a chemistry laboratory on a spacecraft. This method of using the spectrum of light to study the properties of an object is called "Spectroscopy" and the light observed in all its colours is called the spectrum.
Figure 3.1: Absorption lines of Sodium, Nitrogen, Hydrogen and Oxygen, illustration: NASA, ESA, Leah Hustak (STS
Supernova explosion of stars are very short lived events. After the explosion, the brightness reaches a peak and then fades away in brightness. Spectroscopy can be used to know more about these explosions, but it has to be done fast enough before the explosion become faint. When Astrophysicists observed the spectrum from Supernovae explosions. It was seen that a few of them showed weak Hydrogen lines while some showed strong lines. The ones with weak lines were classified as Type 1 (SN1), while the other as Type 2(SN2) \cite{Cosmos}. When they looked at the features in the absorption spectrum of SN1, they noticed that a particular type of Supernovas, which were later called as SN1a showed a substantial decrease in brightness in their later phases. Astrophysicists learnt that this decrease happened due to the energy released by a radioactive $ ^{56}$Ni which has a half life of about 6 days. The $ ^{56}$Ni subsequently produces $ ^{56}$Co which is also radioactive with a half life of about 77 days and decays to $ ^{56}$Fe \cite{Cosmos}. The RCW86 observed by the Chinese astronomers is believed to be SN1a as well\cite{Zhao_2006}.
A star is born out of clouds of gases called Nebula (Figure 1.2 shows the Carina nebula). These gases, which are usually Hydrogen or helium are pulled together by gravity and form clumps. Once there is a lot of gas accumulated at a place gravity pushes the gas together in a way that it forces Nuclear reactions to take place. This reaction produces heat that leads to an increase in pressure of the gas. This pressure pushes the gas outward. However, gravity holds the hot gas inside. A star is therefore simply a ball of gas where there are two forces fighting each other. The pressure of the hot gas trying to burst open the star and the gravitational force holding the star in one piece. There is a delicate balance of outward and inward forces\cite{Cosmos}. \\
Once a star has formed, it starts using the Hydrogen as a nuclear fuel. This Hydrogen is converted to Helium at the center of the star. As the star ages, the newly formed Helium stays at the centre or "core" of the star. After about 10$\%$ of its Hydrogen \cite{Cosmos} is used up, the star starts expanding in size and becomes a red giant. This process is most likely to happen to our Sun too.\\
The next stages of the evolution of the star consists of Helium, Oxygen and Carbon burning. All of these stages need higher and higher temperatures to occur and each phase takes shorter times than the previous phase. At the final stage of evolution of the star, it can either become a white dwarf, neutron star or a black hole. This depends mainly on how much mass a particular star has in its initial stages. If the star had less than 8 times the mass of the sun, then it would most likely become a white dwarf \cite{Cosmos}. If the stars are heavier, they can become a neutron star or a black hole. This also depends on how much mass the star losses in its life time due to various stellar events. If a heavy star looses a lot of mass due to some reason, its last stage could be different.
Figure 3.2: The Carina Nebula, source: ESO
Figure 3.3: Artist’s impression of the hottest and most massive touching double star— ESO/L. Calcada
Since many stars can be formed out of the same Nebula at different positions, we see that sometimes stars are formed very close to each other. They are so close that sometimes they orbit around each other. This phenomenon is called a binary star system. Since stars in the vicinity are all born out of the same cloud, Astrophysicists believe that 85$\% $ of all stars could be in a binary system. The distance between the binary stars play a crucial role in their lifetime. If the stars are very close to each other, there are chances that there could be exchange of material between the two stars.
Supernovas type 1a are believed to be seen in binary system with a white dwarf and a companion star. As the two stars go around each other, sometimes the white dwarf pulls some of the material from the companion star. This process is called accretion. However, each stars can only hold some amount of mass. As we had discussed above, this is a very delicate balance. When we increase the mass of the star, its gravitational force increases, however, the increased mass also increases the pressure that leads to an increase in energy generation.
The maximum mass that can be held by a star is known as the Chandrasekhar mass At a certain time during the accretion, when the mass of the white dwarf reaches about 1.4 times the mass of the sun \cite{10.1007/3-540-15206-7_55} a chain reaction occurs at the intermediate regions of the star, where Oxygen is converted to Calcium. In the outer regions the Carbon and Oxygen layers remain unburnt. This chain reaction releases an immense amount of nuclear and heat energy, so much so that gravity cannot hold the star together. The delicate balance is broken and we get a supernova explosion. \cite{10.1007/3-540-15206-7_55}.
At this juncture, it should be mentioned that this is one hypothesis of how the SN1a explosion happens. There is another model where two binary white dwarfs exchange matter with each other and when the Chandrasekhar mass is reached, there is an explosion. We don't know which one happens for a particular system, what we do know is that the Chandrasekhar mass has to be reached for this explosion to happen. At this mass, the white dwarf become unstable and explodes. This explosion is extremely bright. Some of the brightest objects visible.
We know that the Sn1a goes through a peak brightness and then starts fading. It was also observed that the brighter the supernova, the slower it reduced in brightness. Astrophysicists believe that the freshly exploded white dwarf remnants which now contain a lot of $ ^{56}$Ni undergoes radioactive decay and breaks into $ ^{56}$Co in about 6 days. $^{56}$Co which is also radioactive with a half life of about 77 days subsequently also decays to stable $ ^{56}$Fe. This constant nuclear reaction keeps the brightness of the supernova sustained and gives us a peak. After 17 days, we start observing a decay that is observed from Earth.\\
Each white dwarf has a different amount of $ ^{56}$Ni during explosion. This explains why some of the SN1a explosions had a different peak brightness. Since the peak of brightness of the supernova explosion is strongly dependant on the amount of $ ^{56}$Ni, if we knew how much was present at the time of explosion, we could find out the absolute luminosity of the star \cite{10.1007/3-540-15206-7_55}.
Figure 4.1: A small sample of SN1a and its variation in time
Figure 4.2: The Supernova and its change in brightness ∆M
One way to find out how much $ ^{56}$Ni and other radioactive material was released is to look at the energy produced in the explosion. The more the $ ^{56}$Ni the faster the explosion travels out. However, firstly, the explosion should escape the gravitational force of the white dwarf. The minimum energy needed for this would be $0.5\times 10^51 $ ergs, this is the same as 12 billion trillion trillion tonnes of TNT. This energy be enough to unbind the star, but not enough for an "explosion". The material would just escape the star and stop. For the observed explosion, astrophysicists could look at how fast the material escapes the star. Then calculate how much energy is needed to accelerate this amount of matter at those speeds. We have seen with from RCW86 that the released matter does travel quiet far. The total amount of energy needed to explode a white dwarf like a supernova would be about 36 billion trillion trillion tonnes of TNT\cite{10.1007/3-540-15206-7_55}. This type of explosion is attainable if the star had about 33$\% \textrm{ of } ^{56}$Ni \\
There is another way to do this. We do know that the brightness of the explosion ($M_\textrm{peak}$) is related to how fast the brightness changes. So, this means that there is a relation between the difference in brightness in 15 days($\Delta M$), to the absolute magnitude of the explosion. This would require astrophysicists to measure the peak of the brightness and brightness after 15 days of the peak \cite{1996AJ....112.2438H}. And remember, if we know the absolute magnitude of the explosion and compare it to how bright it appears on Earth, we can know how far the explosion occurred. This method skips the requirement to know how far the released matter travelled and only depends on two pieces of information. "How bright was the supernova during its brightest" and "How much of the brightness did it loose"
Figure 4.3: Absolute magnitude of the SN from Figure 4.1
Looking at figure 4.1, we can see measurements of $\Delta M$ from various supernovas. We can see that the measured brightness for the various Sn1a goes through a peak and then decays are the days pass. The "B" in the figure tells us that a blue filter was attached to the detector. A different filter would have a different brightness curve. Apart from the peak, we can also see that in each of these decay. However, the decay in each of these are different. This is because each of them have different amounts of $ ^{56}$Ni during explosion and as we know, this affects the rate of decay. The difference calculated for each of them after 15 days of the peak is mentioned in Figure 4.2.
Now looking at the Figure 4.3, we can see the same supernovae. But this time, they have been plotted with their Absolute magnitudes. There is clearly a direct correlation observed in its Absolute magnitude and the $\Delta M$. Again, the "B" denotes the blue filter, so everything is consistent. A mathematical relation can then be constructed by scientists to calculate the distance using the Absolute magnitude of the Supernovae.
One of the fundamental questions in Cosmology, the study of the evolution of the Universe is "Where did the Universe come from and where are we going?". To answer this question, scientists look at how other stars and galaxies are moving with respect to us and each other. They make observations to see if galaxies are moving away or coming towards each other. If they are moving away, that means the Universe is probably expanding, while if they are coming towards each other, it means that the Universe is contracting. If there is a net motion of contraction or expansion, is it accelerating or moving at a uniform velocity? There are many questions that need to be answered. When we look up in the sky, stars appear like point like objects in stark darkness. How are we supposed to know if the object is coming towards us, or going away? If this object was similar to the headlight in our earlier example, then we could simply measure the change in brightness. However, stars are not headlights. They do not have the same brightness throughout their life and each star is vastly different from the other. There are also stars that change their brightness frequently. How are we supposed to know if the brightness is changing, or if the star is moving towards us?
We see this in stars too, but instead of change in frequency of sound, we see this in the change in colour of light. For example, if the missing line for Hydrogen in the spectra of a star is observed at a frequency higher or lower than expected, then we can mathematically calculate the relative velocity of the star with respect to Earth. For a SN1a explosion, there is a shift in the peak of the frequency as well, however, since the light from the supernovae changes over time, we can also check for effects of acceleration. This appears as a broadening of the brightness. If the white dwarf was moving away from Earth, the peak would take longer to arrive and be more "stretched out". A nearby SN1a explosion would look like the green curve in Figure 4.4, while one that is moving away from earth would follow the red or the blue curve. The more stretched out the curve is, the faster the system moves away. But what do we mean by "stretched out"
Consider a rubber band that has two points marked on it separated by about 1cm. When you stretch this rubber band and then measure the length of the two points, you will notice that the points are now separated by more than 1cm. This is similar to how you can observe expansion of spacetime. However, this "Expansion" occurs in the time dimension. If you have a flashlight that sends two pulses of light separated by 10 days and the spacetime expands as the pulses travel through space, the gap of 10 days can become 15 days or more, but it cannot remain 10 days. This stretch out, is observed as stretch out of the curve. Since we do know that 85$\%$ of stars are in a binary system, we do expect many of them to undergo a SN1a explosion at some point in their life. Since even distant stars in a completely different galaxy can have a SN1a explosion, we could learn about the distance of this galaxy and how fast it is moving using this . This method of distance measurement is particularly useful because when the explosion occurs, the star can get brighter than the entire host galaxy, therefore, we can observe measure distances to galaxies pretty easily.\\
This is exactly the work that received the 2011 Nobel Prize. The Two teams looked at about 50 distant supernovae. They found that that the supernovae were moving away from each other, however, the remarkable achievement was not only that they were moving away from each other, but that they were moving away faster today than in the past. They observed a stretch out in the curve from the SN1a. Astrophysicists went looking for ways to measure distances using the brightest headlights in the Universe and ended up finding that the Universe is expanding. The "Energy" causing the acceleration of the Universe is still unknown And it is popularly called as "Dark Energy".